The race to create an artificial sun
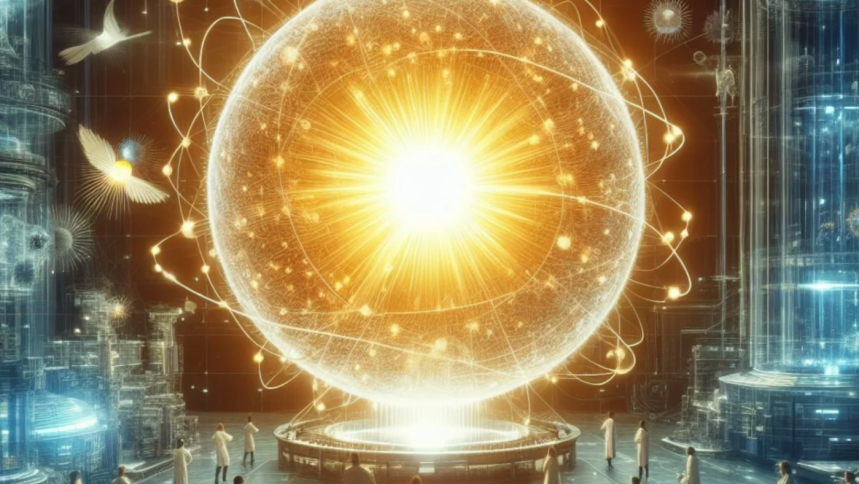
Humans have worshipped the sun since we became sentient. Our journey from ancient civilisations' mystic rituals to today's advanced scientific understanding has only deepened our appreciation for the sun. It has evolved from being the source of light to being the source of all life and energy on planet Earth. The question then arises: can we ever aspire to craft something as sublime or dare to harness its immense power? Remarkably, the answer is yes.
What is nuclear fusion?
At the core of this effort are our breakthroughs in understanding nuclear fusion, the fundamental process powering stars such as our sun to cast light across the universe. Nuclear fusion is the process by which lighter atoms combine into heavier atoms and release energy by converting lost mass into energy. On Earth, we select heavy hydrogen atoms from water as our fusion fuel, presenting a viable path to lessen our reliance on fossil fuels.
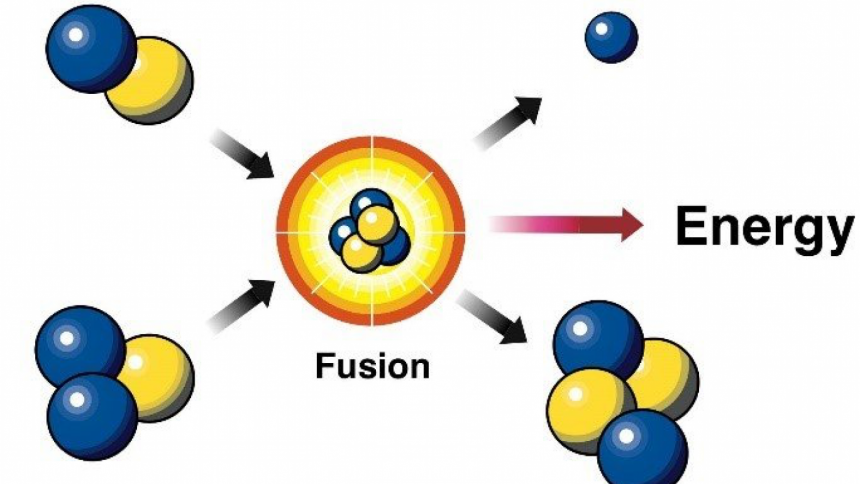
While wind and solar energy are also environmentally friendly, they face challenges in providing steady energy 24/7 for modern living. In contrast, using heavier hydrogen atoms for nuclear fusion promises to provide sustainable energy for millions of years. Importantly, this technology avoids the potentially catastrophic events and radioactive wastes associated with fission reactors like Chernobyl. To note, fission is the process of generating energy via splitting a larger atom into smaller ones, which also releases energy along the way. Any accidents in a fusion reactor result in the fuel slowly fading out without much radioactivity.
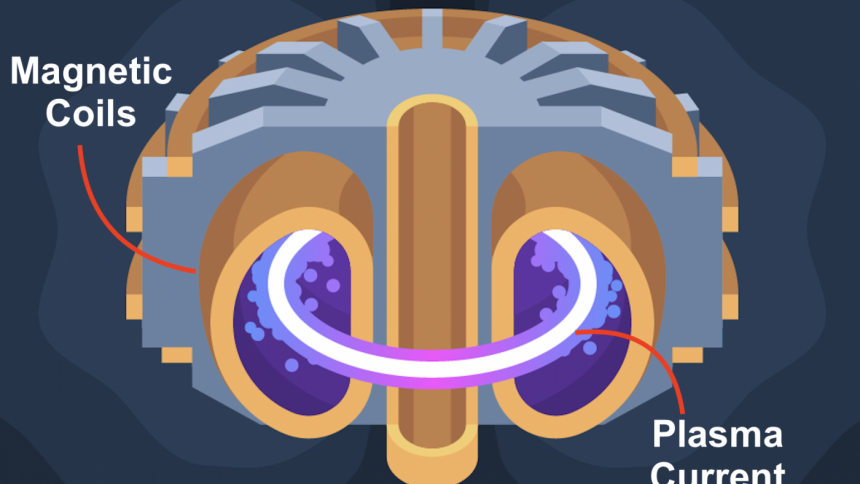
Given these benefits, the Soviet Union and the USA first attempted controlled fusion experiments in the 1950s, creating several prototypes. It quickly became apparent that harnessing fusion energy would require solving two fatal problems. Firstly, atoms must be heated to extreme temperatures (100 million degrees Celsius), turning them into plasma - the fourth state of matter. Secondly, although heating the plasma is feasible, the real challenge is containing this artificial sun made of plasma. The actual sun uses its immense gravity to contain the plasma, which unfortunately we don't have on Earth. Fortunately, there are few promising techniques developed to contain this incredibly hot plasma.
Containing the 'sun'
One approach involves using lasers to simulate conditions inside the sun and simultaneously contain the plasma. Utilising this approach, the National Ignition Facility at Lawrence Livermore National Laboratory has recently achieved a historic milestone by producing the first-ever positive energy gain. Despite this progress, significant hurdles remain for scaling up production.
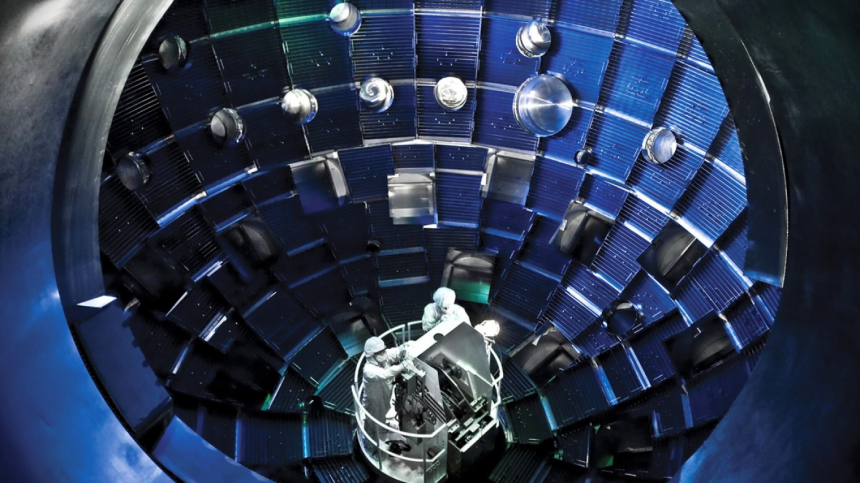
Another technique employs toroidal (donut-shaped) magnetic structures to contain the charged plasma particles. Collaborative efforts among leading global powers—including the USA, China, India, the European Union, Russia, Japan, and Korea—are advancing to make the concept of nuclear fusion reactors a reality.
The International Thermonuclear Experimental Reactor (ITER) is the most ambitious of these collaborations, involving thirty-five countries. This method benefits from the fact that larger devices can dramatically increase energy production. However, the large size comes with the caveat of increased instability.
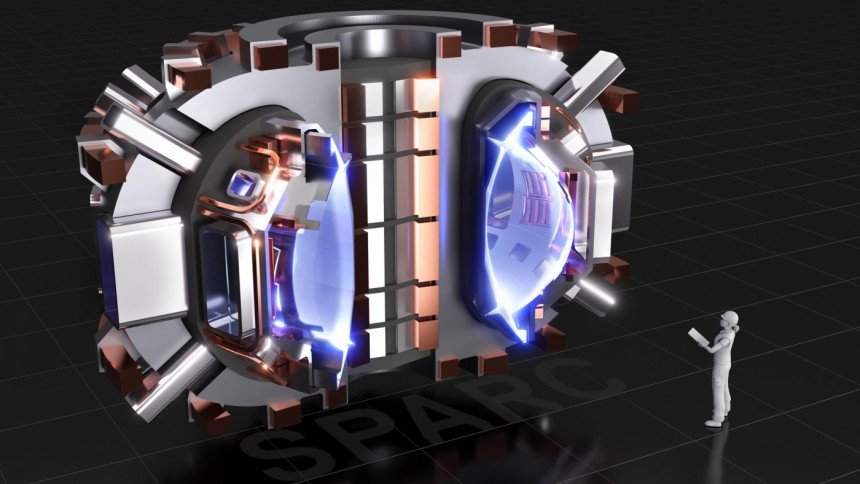
The SPARC tokamak device, a joint effort by Commonwealth Fusion Systems and the Massachusetts Institute of Technology's Plasma Science and Fusion Center (PSFC), uses superconducting magnets to bypass the need for large-scale machinery.
Another compact design, field-reversed configuration (FRC), is gaining attention because of stability and high energy density – e.g., the Princeton Field-Reversed Configuration project at Princeton University's Plasma Physics Laboratory.
This global collaborative ethos in the nuclear fusion research community shines as a beacon of hope for countries like Bangladesh, which may lack the means to pursue an independent fusion program. Such partnerships provide invaluable training for the next generation and position these nations to embrace advanced fusion technology for commercial applications. Despite the obstacles, the promise of a clean, safe, and virtually inexhaustible energy future renders this collective endeavour profoundly meaningful.
Taosif Ahsan is a Physics PhD Candidate at the Massachusetts Institute of Technology.
Comments