THORIUM POWER: YAY OR NAY?
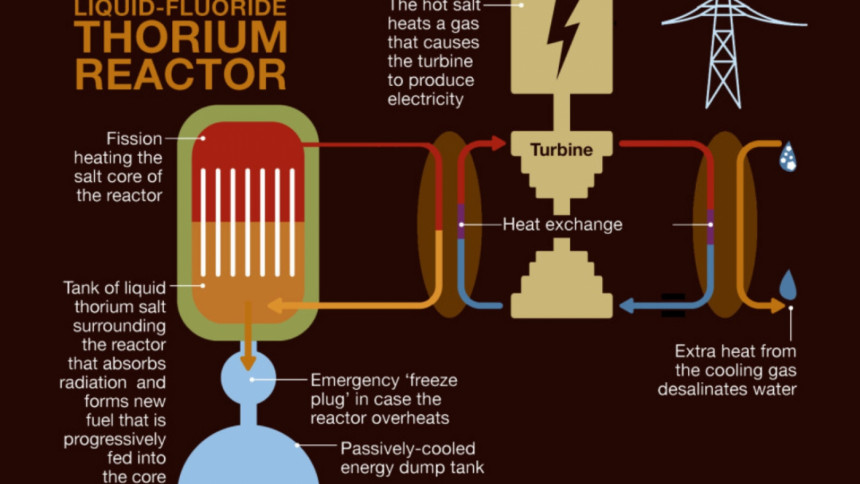
A flying neutron comes charging at an atom. It briefly turns into an unstable atom before decaying into few other atoms and more neutrons. This is nuclear fission in short --- a process that “cleanly” feeds electricity into our lives. These fellow neutrons go on their way to strike more atoms initiating a chain reaction. More heat, more energy. But, when it loses control, this reaction turns itself into a terrific explosion, an uncontrolled chain reaction --- the underlying principle of an atom bomb.
However, the atoms cannot be just any element from the periodic table. Uranium-235 and Plutonium-239 are the ones most commonly used. But what about thorium dwelling in the same row as uranium?
Thorium-232 has been in the spotlight lately as a likely successor of uranium. It is possible for thorium, named after the Norse god, Thor, to power our future by all means. But would we let this thought give birth to reality? The answer is not simple.
Reactors like the Light Water Reactor (LWR) in use today run on U-235. A typical reactor will have a core containing fuel rods, a moderator (made of water or graphite)to slow down electrons, control rods made of boron to absorb neutrons and halt the reaction. This reactor will be flooded by a coolant such as heavy/light water, molten sodium which takes away the heat (preventing a meltdown) and goes to a heat exchanger. Here, water is converted to steam and is sent off to rotating turbines to generate electricity.
A Liquid Fluoride Thorium Reactor (LFTR), pronounced as ‘lifter’, is a type of molten salt reactor, a whole new kind compared to conventional ones. It burns Th-232 to U-233, a fissile isotope. And its suitability to take over can be reasoned in three aspects: safety, fuel efficiency and nuclear waste.
The LFTR has a number of remarkable safety features by default. For instance, they are designed to work at atmospheric pressures, owing to their nature unlike LWR which uses highly pressurised water and runs the risk of explosion. Hence, LWRs require precautionary containment infrastructure. Another safety attribute is to do with “negative temperature coefficient of reactivity.” In layman’s terms, this means that as the reactor’s temperature rises, the reactivity decreases. This means that the reactor is meltdown-proof and the consequences of overheating is not an issue. To reinforce the overall safeness, frozen safety plugs work interestingly. When the reactor core’s temperature exceeds a critical point, this plug melts and drives the liquid fuel into a passively-cooled dump tank thereby effectively cutting off the fuel supply.
Fuel efficiency is key when it comes to building nuclear reactors. In the LWR, there are tonnes of uranium being fed to the reactor. Two hundred and fifty tonnes of uranium contain 1.75 tonnes of U-235. This will undergo an enrichment process and finally there will only be 35 tonnes left, out of which 1.15 tonnes is U-235.
When burned, not much changes. The same 35 tonnes except 33.4 tonnes constitutes U-238, 0.3 tonnes of U-235, 1 ton of fission by-products and lastly, 0.3 tonnes of “useful” plutonium. This plutonium was the very reason why research on thorium was aborted as uranium yields to what can be used to manufacture nuclear weapons. Given the turmoil of the Cold War era at the time, this proved to be a “safer” option to a number of nations.
But, bring in thorium and the calculation changes entirely. One tonne of thorium burns completely to give the same amount of fission by-products. Moreover, within 10 years, 83 percent of these will be stable. The rest 17 percent needs to be stored away for about 300 years. This is due to the different spectrum of by-products formed. A very small amount of plutonium is also formed which was frowned upon initially. The heat and radiation damages a significant portion of the uranium fuel rods, requiring them to be taken out before being completely burnt. Thus, a tonne of natural thorium produces as much energy as 35 tonnes of enriched uranium on average. To top it off, thorium is 3 times more abundant than uranium in the earth’s crust.
But if thorium really is that great, why not pursue it?
Because at the moment the nuclear industry is lacking operational experience with the system. The only time thorium was used in the reactors was after the World War II at the Oak Ridge National Laboratory way back in the 1960s.
There are concerns regarding commercial viability as it remains unproven on a commercial scale. Thorium is not ideally suited for current reactors and hence new designs have to be adapted. All this begets the question of sufficient funding. Some experts even claim thorium power to be nothing but a distraction.
The hype is lesser radioactive waste, virtually no weapons-grade by-products and cheaper fuel. Despite the odds, climate change is taking over the world ruthlessly. To combat carbon emissions and recover from all the damage caused already, nuclear energy is deemed to be one of the fastest ways to do so. It is high time we prioritise where we pump our money.
Hiya loves food that you hate by norm- broccoli, pineapple pizza and Bounty bars. Find her at hiyaislam.11@gmail
Comments